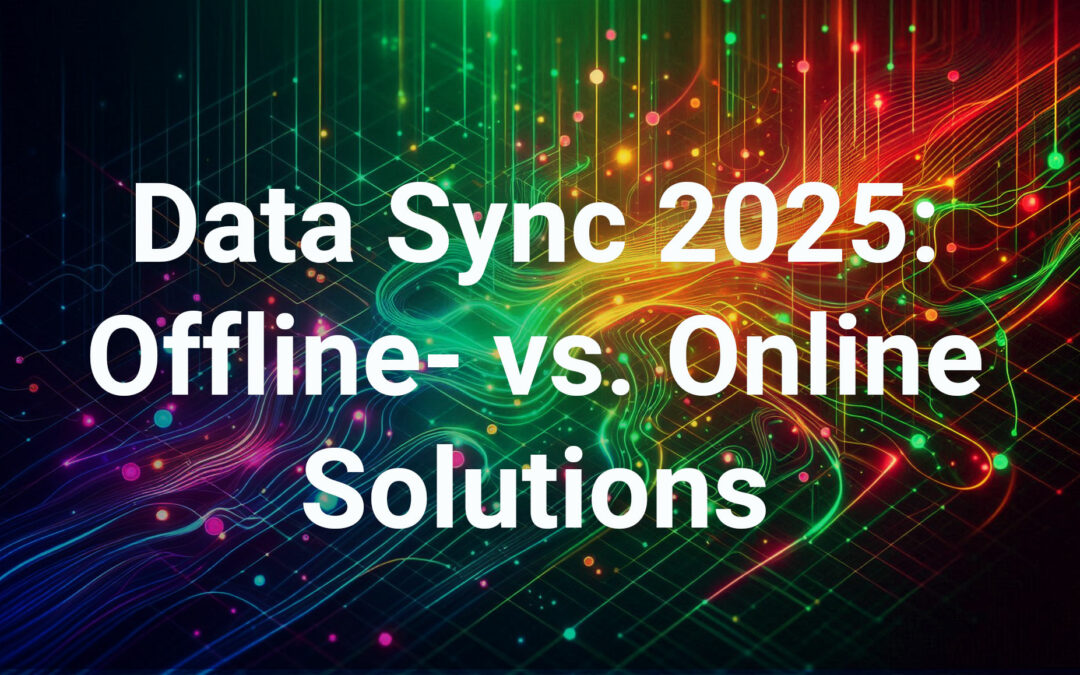
Data Sync Alternatives: Offline vs. Online Solutions
Ever waited to order or pay with a waiter holding their ordering device in the air for a signal? These moments show why offline-first Data Sync is essential. With more and more services relying on the availability of on-device apps and the IoT market projected to hit $1.1 trillion by 2026, choosing the right solution – particularly online-only or offline-first data sync – is more crucial than ever. In this blog, we discuss their differences and highlight common Data Sync alternatives.
What is Data Sync?
Data synchronization (Sync) aligns data between two or more devices to maintain consistency over time. It is an essential component in applications ranging from IoT and mobile apps to cloud computing. Challenges in data synchronization include asynchrony, conflicts, and managing data across flaky networks.
Data Sync vs. Data Replication
Data Synchronization is often confused with Data Replication. Nevertheless, they serve different purposes:
- Data Replication: A unidirectional process (works in one direction only) that duplicates data across storage locations to ensure availability and prevent loss. It is simple but limited in its application, and efficiency, and lacks conflict management.
- Data Synchronization: A bidirectional process that harmonizes all or a subset of data between two or more devices. It ensures consistency across devices and entails conflict resolution. It is inherently more complex but also more flexible.
Online vs Offline Solutions: Why Offline Sync Matters
Online-only synchronization solutions rely entirely on cloud infrastructure, requiring a stable internet connection to function. While these tools offer simplicity and scalability, their dependency on constant cloud connectivity brings limitations: Online Data Sync solutions cannot guarantee response rates and their speed varies depending on the network. They do not work when offline or in on-premise settings. Using an Online Sync solution often entails sharing the data and might not comply with data privacy requirements. So, do read the terms and conditions.
Offline-first solutions (offline Sync) focus on local data storage and processing, ensuring the app remains fully functional even without an internet connection. When a network is available, the app synchronizes seamlessly with a server, the cloud, or other devices as needed. These solutions are ideal for on-premise scenarios with unreliable or no internet access, mission-critical applications that must always operate, real-time and high-performance use cases, as well as situations requiring high data privacy and data security compliance.
A less discussed, but in our view also relevant point, is sustainability. While there might be exceptions depending on the use case, for most applications offline-first solutions are more resourceful and therefore more sustainable. If CO2 footprint or battery usage is of concern to you, you might want to look into offline-first Data Sync alternatives.
Now, let’s have a look at current options:
Data Sync Alternatives
(If you are on mobile, click here for a view that’s optimized for mobile)
Solution | Company | Type | Offline Support | Self-hosted Sync | Decentralized Sync | Database | Type of DB | OS/Platforms | Languages | Open-Source Component | License | Other Considerations | Country |
Firebase | Google (Firebase was acquired by Google in 2014) |
Online | Local cache only, no persistence, syncs when online | ❌ | ❌ | Cloud: Firebase Realtime Database; Edge: Only caching, no DB (called Firestore) | Document store | iOS, Android, Web | Java JavaScript Objective-C Swift Kotlin C++ Dart C# Python, Go, Node.js |
❌ | proprietory | Tied to Google Cloud, requires internet connectivity | 🇺🇸 |
Supabase | Supabase | Online | Limited | ✅ | ❌ | Cloud DB: PostgreSQL | Relational document store | Primarily a cloud solution | JavaScript/TypeScript Flutter/Dart C# Swift Kotlin Python |
✅ | Apache License 2.0 | Supabase is mainly designed as a SaaS, for use cases with constant connectivity | 🇸🇬 |
ObjectBox Sync | ObjectBox | Offline-first | ✅ | ✅ | In development | ObjectBox | Object-oriented embedded NoSQL DB | Android, Linux, Ubuntu, Windows, macOS, iOS, QNX, Raspbian, any POSIX system really, any cloud (e.g. AWS/Azure/Google Cloud), bare metal |
C C++ Java Kotlin Swift Go Flutter / Dart Python |
✅ | DB: Open source bindings, Apache 2.0, closed core | Highly efficient (saves CPU, Memory, battery, and bandwidth); fully offline-first, supports on-premise settings, 100% cloud optional | 🇩🇪 |
Couchbase (Lite + Couchbase Sync Gateway) | Couchbase (a merger of Couch One and Membase) | Online | ✅ | The CE Sync is a bare minimum and typically not usable; Self-hosted Sync with Couchbase Servers is available as part of their Enterprise offering | ✅ as part of the Enterprise offering; gets expensive quickly | Edge: Couchbase Lite; Server: Couchbase | Multi-model NoSQL document-oriented database | Couchbase Lite: iOS, Android, macOS, Linux, Windows, Raspbian
and Raspberry Pi OS Couchbase Sync Gateway: Red Hat Enterprise Linux (RHEL) 9.x, Alma Linux 9.x, Rocky Linux 9.x, Ubuntu, Debian (11.x, 12.x), Windows Server 2022 |
.Net C Go Java JavaScript info Kotlin PHP Python Ruby Scala |
✅ | Couchbase Lite is available under different licenses; the open source Community Edition does not get regular updates and misses many features especially around Sync (e.g. it does not include Delta Sync making it slow and expensive) | Typically requires Couchbase servers, quickly gets expensive | 🇺🇸 |
MongoDB Realm + Atlas Device Sync | MongoDB (Realm was acquired by MongoDB in 2019) |
Offline-First | ✅ | Cloud-based sync only | ❌ | Cloud: MongoDB, Edge: Mongo Realm DB | MongoDB: NoSQL document store; RealmDB: Embedded NoSQL DB | MongoDB: Linux OS X Solaris Windows Mongo Realm DB: Android, iOS |
more than 20 languages, e.g. Java, C, C#, C++ | ✅ | MongoDB changed its license from open source (AGPL) to MongoDB Inc.’s Server Side Public License (SSPL) in 2018. RealmDB is open source under the Apache 2.0 License. The Data Sync was proprietary. | Deprecated (in Sep 2024); End-of-life in Sep 2025; ObjectBox offers a migration option | 🇺🇸 |